The role of convection in mid-latitude cyclones
Even “slowly ascending” warm conveyor belts have their convective moments.
18.08.2016
Cyclones, also called low-pressure systems, are responsible for most of the weather in the mid-latitudes. Around half of the precipitation over Central Europe, for example, is directly associated with a cyclone. This fraction is even larger in winter. The formation of rain droplets is caused by lifting, and the associated cooling, of air. In a cyclone, the lifting mostly occurs at the cold front, a barrier between the warmer air, usually to the South-East, and colder, polar air from the North-West. The mechanism of this lifting, however, varies drastically from cyclone to cyclone. In winter, cold fronts are often stretched out over several thousand kilometers, and air gradually ascends parallel to the front as it moves from the sub-tropics to the polar regions. Such air streams are often called warm conveyor belts (see figure below). In summer, on the other hand, when the atmosphere is unstable, cold fronts can trigger narrow lines of convection (also called thunderstorms) with rapidly ascending air.
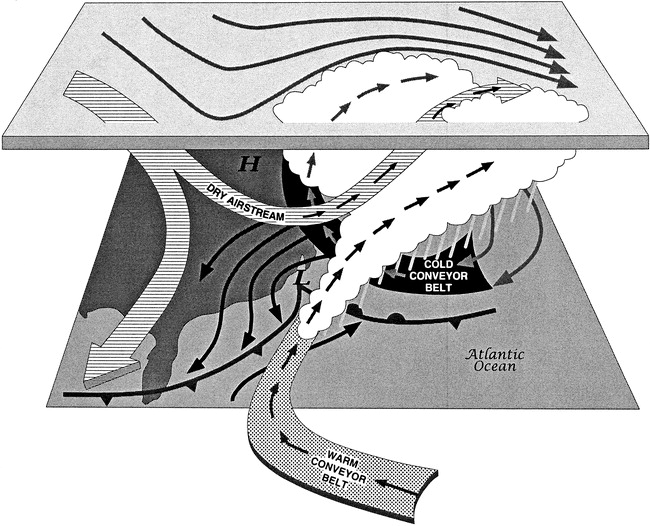
A schematic depiction of the conveyor belt model1. The fronts belonging to the low-pressure system (L) are shown at the surface in their common notation. The warm conveyor belt starts out in the South and then ascends parallel to the cold front, thereby producing clouds and rain.
The goal of a study by Stephan Rasp, Tobias Selz and George Craig of the theoretical meteorology group at MIM, which has just been published in the journal Monthly Weather Review, was to use cutting-edge computing tools to investigate the difference in the aforementioned types of air mass ascent. The authors used a novel tool to trace several hundred thousand air parcels in their numerical weather model simulations of three cyclones in the Euro-Atlantic region. The case studies were chosen to represent different regimes and seasons as shown in the figure below. In the winter case (JAN) ascent occurs in a warm conveyor belt which extends several thousand kilometers from the sub-tropical Atlantic West of the Maroccan coast to Ireland. The summer case (JUL) is an example of fast convective ascent in a line of heavy thunderstorms over France and Germany. In the autumn case (OCT), both ascent types are present over the Mediterranean. The simulations had a high enough resolution to be able to represent deep convection, an important advance compared to previous studies.
The animations above show the simulations of the three cases which were chosen for this study. JAN is the winter case, JUL the summer case and OCT the autumn case. The elongated lines of precipitation (blue shading) coincide with the locations of the cold front, along which the tracked air parcels (dots, colored by their pressure) ascend. Also shown is the convective available potential energy (CAPE), a measure of how unstable the atmosphere is, in the red shading. The thin black lines show the geopotential height contours.
The first aim of this study was to investigate whether the simulation data clearly shows the difference in the ascent characteristics of the three cases. Indeed, the results suggest that air parcels which ascend in unstable regions of the atmosphere, for example in summer, typically rise about ten times as fast as parcels in winter. This finding confirmed the picture of a clear scale separation between synoptic and convective ascent which has been proposed by previous studies. The catch, however, is that even air parcels which need a long time to travel all the way from the boundary layer, the lower 1 km of the atmosphere, to the tropopause region can experience phases of very fast lifting (see figure below). The mechanisms for this phenomenon differ in the investigated cyclones. In the winter case a low-level convergence line at the cold front fuelled a process called line convection, while in the autumn case the embedded phase of rapid ascent was located higher up in the free atmosphere due to a layer of unstable air. These findings could have implications for the understanding of forecast-error growth, or in other words, the degradation of forecast quality with time. Studies have shown that errors grow especially fast in regions of fast vertical ascent, which can then completely ruin a forecast within a few days, a phenomenon often called “forecast bust”. Therefore, the degree of convective activity in a cyclonic system might matter a lot when it comes to how predictable weather is. Warm conveyor belts which contain a significant amount of convective ascent could therefore potentially lead to such “forecast busts”.
This figure shows ten randomly selected air parcel trajectories for the summer case (JUL, on the left) and the winter case (JAN, on the right). The convective summer trajectories show a rapid lifting from the near-surface (1000-900 hPa) to just below the tropopause (400-200 hPa). The non-convective winter parcels, on the other hand, ascend more slowly on average but many still have bursts of embedded fast ascent (see black arrow).
The second major result of this study concerns the impact of the rising air on the dynamics of the atmosphere. Meteorologists often define a quantity called potential vorticity (PV) which is a combined measure of the vertical structure of the atmosphere and the rotation of an air parcel. This quantity has turned out to be particularly useful because, under “normal” conditions, i.e. far away from any rain, an air parcel will always maintain its PV. In other words, PV is conserved following an air parcel. This is one of the properties which makes PV a powerful tool for theoretical meteorologists. It can, for example, be used to track the origin of a dynamically important air mass, or to understand the impact of processes which change the PV on the dynamics of the atmosphere. One such PV-modifying process is the release of heat by condensation and freezing, associated with the lifting of air. As the air ascends, its PV first increases and then decreases as a result of these phase transitions (see figure below). The resulting local PV maximum acts to strengthen the cyclone. Strangely enough, since there is no dynamical explanation for why it should be this way, the mean PV values of an air stream before and after the ascent have been found to be almost identical in all previous studies which were conducted with lower-resolution models. Therefore, the authors were curious to see if their high-resolution simulations produced similar results. As shown in the figure below, the similarity between mean inflow and outflow PV is remarkable, regardless of the differing characteristics of each case. This supports previous findings and hints at a not yet fully understood fundamental behaviour of the atmosphere.
The figure above shows the evolution of the mean potential vorticity (PV) for the three cases, where in the autumn case the parcels have been separated into convective and non-convective parcels (OCTc and OCTnc). The time axis is centered relative to the midpoint of the ascent (t = 0). The PV peak at the time of the ascent is clearly visible, and is much sharper for the convective cases (JUL and OCTc). All four lines, however, show a remarkable similarity of the mean PV value before and after the ascent.
This study was partly conducted within the new DFG-research project Waves to Weather, in which scientists from several institutions in Southern Germany are investigate further the effect of ascending air, and the associated physical processes, on the growth of forecast errors. The aim is to gain a better understanding of which processes represent a significant source of uncertainty for weather forecasts and how these uncertainties can be adequately represented in numerical weather models. Further investigation is needed to fully understand many of these complex processes. This study represents one step forward to gain a better understanding of the atmosphere.
References:
1. Kocin, P.J. and Uccellini, L.W., 1990. Snowstorms along the northeastern coast of the United States, 1955 to 1985 (Vol. 22). American Meteorological Society.